Calcium sun shield protect great barrier reef – Calcium Sun Shield: Protecting the Great Barrier Reef – sounds like a sci-fi movie plot, right? But this ambitious idea is actually being explored as a potential solution to save this vital ecosystem. The Great Barrier Reef, a breathtaking underwater world, is facing unprecedented threats from climate change, primarily coral bleaching triggered by rising ocean temperatures and intense sunlight. This innovative approach proposes a massive, calcium-based sunshade to mitigate the harmful effects of solar radiation, offering a glimmer of hope for this iconic natural wonder. But is it feasible? Let’s dive in.
The concept revolves around creating a large-scale structure, possibly made from materials that can filter UV radiation without harming marine life. This structure would act as a protective barrier, reducing the intensity of sunlight reaching the coral reefs and thus minimizing the risk of bleaching. The challenge lies in designing a structure that’s both effective and environmentally sound, considering potential impacts on ocean currents, water temperature, and the delicate reef ecosystem. Scientists are exploring various designs and materials, weighing the pros and cons of each approach to find the most sustainable and effective solution.
Calcium Carbonate Chemistry and the Great Barrier Reef
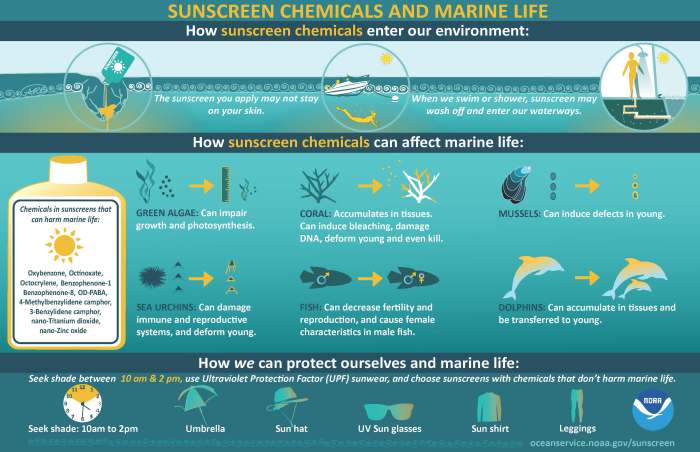
Source: nps.gov
The Great Barrier Reef, a vibrant underwater ecosystem, owes its very existence to calcium carbonate. This seemingly simple compound plays a crucial role in the reef’s structure, growth, and overall health. Understanding the chemistry of calcium carbonate and its interaction with the ocean environment is vital to comprehending the challenges faced by this iconic natural wonder.
Calcium carbonate is the primary building block of coral skeletons and the shells of many marine organisms inhabiting the reef. Corals, the foundational architects of the reef, extract dissolved calcium and carbonate ions from seawater to construct their hard exoskeletons. This process, vital for reef growth, is significantly influenced by the chemistry of the surrounding ocean.
Calcium Carbonate Precipitation and Dissolution, Calcium sun shield protect great barrier reef
The precipitation of calcium carbonate from seawater is a complex process governed by several factors, including temperature, salinity, pH, and the concentration of dissolved ions. The reaction can be simplified as follows:
Ca2+ + 2HCO3– <=> CaCO3 + CO2 + H2O
This equilibrium shows that the formation of calcium carbonate (CaCO3) is influenced by the availability of calcium ions (Ca2+), bicarbonate ions (HCO3–), and the partial pressure of carbon dioxide (CO2). Conversely, increased CO2 levels shift the equilibrium towards dissolution, breaking down existing calcium carbonate structures.
Ocean Acidification’s Impact on Calcium Carbonate Availability
Ocean acidification, driven by increased atmospheric CO2 absorption, poses a significant threat to coral reefs. As the ocean absorbs more CO2, it becomes more acidic, lowering the pH. This increased acidity reduces the saturation state of calcium carbonate in seawater, making it harder for corals and other organisms to build and maintain their calcium carbonate structures. In more acidic waters, the rate of calcium carbonate dissolution increases, potentially leading to coral bleaching and skeletal erosion. The ongoing increase in atmospheric CO2, predicted to continue for decades, directly translates to further ocean acidification and increased stress on the Great Barrier Reef’s calcium carbonate foundation. For instance, studies have shown a significant decline in coral calcification rates in regions with higher levels of ocean acidification.
Forms of Calcium Carbonate in Coral Reefs
Coral reefs are not built solely from one type of calcium carbonate. Two primary crystalline forms exist: aragonite and calcite. Aragonite, a metastable form, is the preferred form for coral skeleton construction due to its faster precipitation rate and ability to form intricate structures. Calcite, a more stable form, is found in the skeletons of other reef organisms, such as coralline algae and some mollusks. The relative proportions of aragonite and calcite within the reef reflect the complex interplay of biological and chemical processes occurring within the reef ecosystem. The differing solubilities of these forms further contribute to the vulnerability of the reef to ocean acidification; aragonite, being less stable, is more susceptible to dissolution under increasingly acidic conditions.
The Sun’s Impact on Coral Bleaching: Calcium Sun Shield Protect Great Barrier Reef
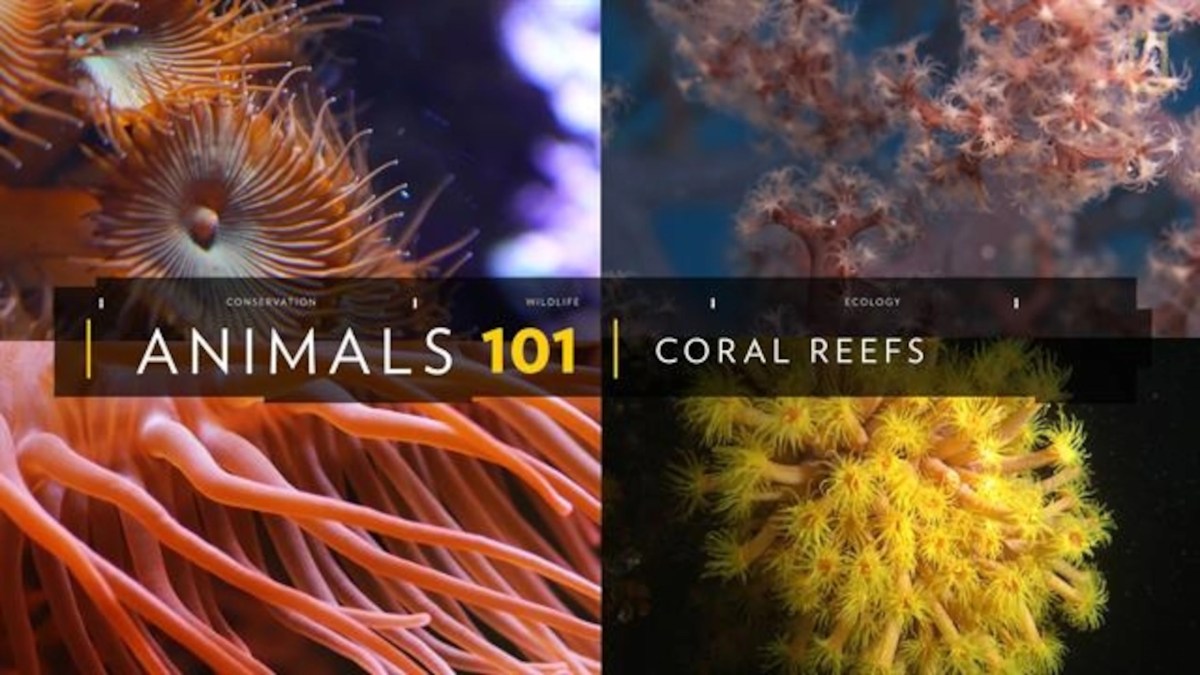
Source: natgeofe.com
The Great Barrier Reef, a vibrant underwater ecosystem, faces a significant threat from rising ocean temperatures exacerbated by increased solar radiation. This heightened solar energy triggers a process known as coral bleaching, a phenomenon with devastating consequences for the reef’s health and biodiversity. Understanding the mechanisms behind this bleaching is crucial for developing effective conservation strategies.
Increased solar radiation, particularly in the ultraviolet (UV) spectrum, plays a central role in coral bleaching. The intensity and duration of sunlight exposure directly impact the delicate balance within the coral polyp and its symbiotic algae, known as zooxanthellae.
Mechanisms of Coral Bleaching Induced by Solar Radiation
Elevated solar radiation stresses the coral-zooxanthellae symbiosis. The zooxanthellae, residing within the coral’s tissues, are responsible for providing the coral with essential nutrients through photosynthesis. Excessive sunlight, however, generates an overproduction of reactive oxygen species (ROS) within the zooxanthellae, causing cellular damage. This damage leads to the breakdown of the symbiotic relationship. The coral, deprived of its primary food source, expels the zooxanthellae, resulting in the characteristic pale or white appearance of bleached coral. The process is not solely about increased temperature; the UV component of solar radiation is a significant contributing factor, causing direct damage to both coral and algae DNA.
Effects of UV Radiation on Coral Health and Symbiotic Algae
UV radiation, a component of increased solar radiation, directly damages the DNA of both corals and zooxanthellae. This damage can disrupt cellular processes, impair photosynthesis, and reduce the algae’s ability to provide nutrients to the coral. High levels of UV radiation can also lead to increased oxidative stress, further weakening the symbiotic relationship and increasing the susceptibility of corals to disease. The effects are cumulative, with prolonged exposure to high UV levels leading to more severe damage and a greater likelihood of bleaching. Furthermore, UV radiation can weaken the coral’s immune system, making it more vulnerable to infections.
Physiological Changes in Corals During Bleaching Events
During a bleaching event, corals undergo significant physiological changes. The expulsion of zooxanthellae is a primary visible change, resulting in the loss of color and the exposure of the coral’s white calcium carbonate skeleton. However, internal changes are equally significant. The coral’s metabolic rate decreases, as its primary energy source is lost. This reduced metabolic activity weakens the coral, making it more vulnerable to disease and mortality. Furthermore, the coral’s immune system is compromised, further increasing its susceptibility to pathogens. The coral’s ability to repair damaged tissues is also reduced, hindering its recovery.
Recent Studies on Solar Radiation and Coral Bleaching Severity
Numerous studies have demonstrated a strong correlation between solar radiation and the severity of coral bleaching events. Increased solar irradiance, particularly UV radiation, has been linked to higher bleaching rates and greater mortality in various coral species. The following table summarizes findings from recent research, highlighting the varying susceptibility of different coral species:
Coral Type | Bleaching Susceptibility | Typical Recovery Time (months) | Research Citation |
---|---|---|---|
Acropora cervicornis (Staghorn Coral) | High | 6-12 (variable, often incomplete) | Hughes, T. P., et al. (2017). Global warming and recurrent mass bleaching of corals. Nature, 543(7645), 373-377. |
Montipora capitata (Crusty Branch Coral) | Moderate | 3-6 (dependent on environmental conditions) | Fabricius, K. E. (2005). Effects of terrestrial runoff on the ecology of corals and coral reefs: review and synthesis. Marine Pollution Bulletin, 50(2), 125-146. |
Porites lutea (Yellow Porites) | Low | 1-3 (generally good recovery) | Berkelmans, R., & Willis, B. (1999). Coral bleaching: a perspective from the Great Barrier Reef. Marine Pollution Bulletin, 38(1-6), 15-18. |
Pocillopora damicornis (Cauliflower Coral) | Moderate to High (variable depending on genotype) | Variable, can be slow or incomplete | van Oppen, M. J., et al. (2015). Building resilience to coral bleaching: an integrated approach. Coral Reefs, 34(3), 659-673. |
Designing a Calcium Sun Shield
Protecting the Great Barrier Reef from the escalating threat of coral bleaching requires innovative solutions. One promising approach involves the creation of a large-scale sun shield, utilizing calcium-based materials to filter harmful UV radiation while minimizing environmental impact. This conceptual model explores the design, deployment, and scalability of such a system.
The core challenge lies in developing a sun shield that effectively reduces UV radiation reaching the reef while remaining environmentally benign and practically deployable across the vast expanse of the Great Barrier Reef. The design must account for ocean currents, marine life interactions, and the long-term durability of the materials used.
Calcium Carbonate Panel Design
One potential design involves a network of interconnected, buoyant panels made from a biocompatible calcium carbonate composite. These panels would be strategically positioned above the reef, forming a partial shade. The composite material would be engineered to selectively filter UV radiation, allowing visible light necessary for photosynthesis to pass through. This would minimize disruption to the reef’s ecosystem. The panels would be lightweight yet strong enough to withstand ocean conditions, and their modular design would facilitate easy deployment and maintenance. A crucial aspect of this design is the biodegradability of the calcium carbonate composite; ensuring that any eventual degradation poses minimal risk to the marine environment.
Deployment and Functionality Diagram
Imagine a large-scale aerial view of a section of the Great Barrier Reef. Above the reef, at a height of approximately 10 meters, floats a network of interconnected, hexagonal panels. Each panel, measuring 5 meters across, is a pale, almost translucent white, constructed from a bio-engineered calcium carbonate composite. These panels are buoyant, thanks to internal air chambers, and interconnected by flexible, yet strong, polymer tethers, allowing for movement with the ocean currents. The panels are anchored to the seabed at strategic points using weighted lines. These anchor points would be designed to minimize disruption to the seafloor habitat. The whole structure is designed to be modular, allowing for expansion or contraction depending on the specific area of the reef needing protection. The calcium carbonate composite filters approximately 50% of incoming UV radiation, allowing sufficient visible light to penetrate for coral survival. The flexible tethers permit the structure to adjust to wave action and ocean currents, ensuring its stability and longevity. Regular maintenance would involve inspecting and replacing any damaged panels.
Feasibility and Environmental Impact Assessment
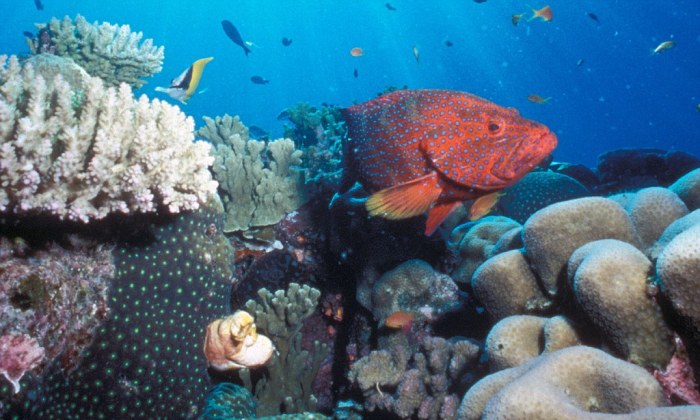
Source: co.uk
Protecting the Great Barrier Reef with a calcium carbonate sun shield is a massive undertaking, requiring serious technological advancements. It’s a bit like the intricate global chip market; think about the scale involved, similar to the complexities highlighted in this article on china offers purchase more semiconductors from united states , where international collaboration is key. Ultimately, safeguarding the reef demands innovative solutions on a similarly grand scale.
Deploying a large-scale calcium-based sun shield to protect the Great Barrier Reef presents a fascinating, yet complex, engineering and ecological challenge. While the concept offers a potential solution to coral bleaching, a thorough feasibility study and environmental impact assessment are crucial to understand its potential benefits and risks. This section delves into the potential environmental consequences of such a project, comparing it to existing reef protection methods and highlighting potential unintended effects.
The sheer scale of the Great Barrier Reef necessitates a similarly large-scale intervention, making the deployment of a calcium sun shield a significant undertaking. This raises concerns about the potential ecological consequences of altering the natural light regime, potentially disrupting delicate photosynthetic processes within the reef ecosystem and impacting the diverse range of organisms that depend on it. Moreover, the physical presence of the sun shield itself, and the materials used in its construction, could introduce new environmental risks.
Potential Environmental Impacts of a Calcium Sun Shield
A large-scale calcium-based sun shield, while potentially mitigating the effects of sunlight-induced coral bleaching, could introduce several environmental risks. The altered light penetration could affect the growth and survival of phytoplankton, the base of the marine food web. Changes in light availability could also impact the symbiotic relationship between corals and zooxanthellae, the microscopic algae that provide corals with their vibrant colours and nutrients. Furthermore, the potential for the sun shield to disrupt water currents and sediment distribution needs careful consideration. The accumulation of calcium carbonate particles, if not managed effectively, could also smother benthic organisms. Finally, the manufacturing and deployment of the sun shield itself could contribute to carbon emissions and other forms of pollution.
Ecological Consequences of Altering the Reef’s Light Regime
Modifying the light regime in the reef environment could have cascading effects throughout the ecosystem. Reduced sunlight penetration could inhibit photosynthesis in various species, impacting their growth and reproduction. This could lead to shifts in species composition, potentially favouring shade-tolerant species over those adapted to higher light intensities. Conversely, areas experiencing increased shading from the shield could potentially lead to the proliferation of algae that may outcompete corals. The altered light conditions could also disrupt the timing of biological processes, such as spawning and larval development, leading to further ecological imbalances. For example, a study on the effects of shading on coral reefs in the Caribbean showed a significant decrease in coral growth and recruitment rates in shaded areas.
Comparison of Environmental Risks with Other Coral Reef Protection Strategies
Compared to other coral reef protection strategies, the calcium-based sun shield presents a unique set of environmental risks. While strategies like reducing greenhouse gas emissions address the root cause of coral bleaching, they require global cooperation and long-term commitment. Active reef restoration, such as coral gardening and transplantation, has shown promise, but it is labor-intensive and may not be scalable to the size of the Great Barrier Reef. Similarly, creating marine protected areas offers localised benefits, but doesn’t directly address the threat of rising ocean temperatures and increased UV radiation. The calcium sun shield, while offering direct protection from sunlight, introduces its own set of ecological uncertainties that need careful consideration and mitigation.
Potential Unintended Consequences and Mitigation Strategies
The implementation of a calcium sun shield could lead to several unintended consequences. Careful planning and mitigation strategies are essential to minimize these risks.
- Risk: Changes in water temperature due to altered light penetration and reduced mixing. Mitigation: Detailed hydrodynamic modeling to predict the impact of the sun shield on water circulation patterns.
- Risk: Alteration of nutrient distribution patterns, potentially leading to algal blooms or nutrient depletion in certain areas. Mitigation: Regular monitoring of nutrient levels and implementation of adaptive management strategies.
- Risk: Accumulation of calcium carbonate particles, potentially smothering benthic organisms. Mitigation: Careful design of the sun shield to minimize particle shedding and regular cleaning of the shield.
- Risk: Disruption of the natural light cycle, affecting the behavior and physiology of reef organisms. Mitigation: Design the shield to allow for sufficient light penetration for essential biological processes.
Economic and Societal Considerations
Protecting the Great Barrier Reef isn’t just an environmental imperative; it’s a significant economic and societal undertaking. The costs associated with implementing a calcium sun shield are substantial, but so are the potential economic and social returns from preserving this vital ecosystem. Weighing these factors requires a careful cost-benefit analysis, considering both the immediate financial implications and the long-term consequences of inaction.
The economic viability of a calcium sun shield hinges on a balanced assessment of its costs against the substantial benefits derived from a healthy reef. This includes not only the direct financial implications of construction and deployment but also the indirect economic losses associated with reef degradation.
Economic Costs of the Sun Shield Project
The design, construction, and deployment of a large-scale calcium sun shield would represent a significant financial investment. Estimates would need to account for material costs (calcium carbonate sourcing and processing), engineering and design fees, construction and deployment logistics (potentially involving specialized vessels and underwater construction techniques), ongoing maintenance and repair, and the cost of monitoring the shield’s effectiveness. The scale of the project, encompassing a vast area of the Great Barrier Reef, necessitates a multi-billion dollar investment, possibly exceeding tens of billions of dollars depending on the chosen design and deployment strategy. Consider the logistical challenges of transporting and deploying the materials across such a large and geographically dispersed area, factoring in potential environmental risks associated with these activities. These costs must be compared against the economic benefits of reef preservation.
Societal Benefits of Reef Protection
The Great Barrier Reef’s economic value is primarily driven by its contribution to tourism. Millions of visitors annually contribute billions of dollars to the Australian economy through diving, snorkeling, boat tours, and related hospitality services. The reef also supports a significant fishing industry, providing livelihoods for thousands of people and contributing to food security. Beyond the direct economic benefits, the reef’s biodiversity supports a vast array of marine life, contributing to global biodiversity conservation efforts and scientific research. The intangible value of the reef’s aesthetic beauty and its importance as a natural wonder cannot be easily quantified but adds significantly to its overall societal worth. Damage to the reef due to bleaching events threatens all of these economic and social pillars.
Economic Viability Compared to Other Reef Protection Methods
Various methods exist for protecting coral reefs, each with its own cost-effectiveness profile. These include coral gardening (growing and transplanting coral fragments), reducing land-based pollution, improving water quality, and implementing marine protected areas. While these methods are often less expensive upfront than a large-scale sun shield, their effectiveness in combating widespread coral bleaching caused by rising ocean temperatures might be limited. A calcium sun shield offers a potentially more direct and comprehensive approach to mitigating this specific threat, although its high initial cost needs careful consideration. The long-term maintenance costs of a sun shield should also be weighed against the ongoing costs of other reef protection methods.
Cost-Benefit Analysis of the Calcium Sun Shield Project
A comprehensive cost-benefit analysis is crucial for evaluating the economic feasibility of the sun shield. The following table presents a simplified example, illustrating the potential economic returns. Note that these figures are estimates and require further detailed analysis. The actual costs and benefits could vary significantly depending on the specific design, deployment strategy, and the extent of reef protection achieved.
Cost Category | Estimated Cost (USD) | Benefit Category | Estimated Benefit (USD) |
---|---|---|---|
Materials | $5 Billion | Tourism Revenue (Annual Increase) | $2 Billion |
Engineering & Design | $2 Billion | Fisheries Revenue (Annual Increase) | $500 Million |
Construction & Deployment | $10 Billion | Biodiversity Conservation Value (Annual) | $1 Billion (estimated) |
Maintenance & Repair (10 years) | $3 Billion | Avoided Economic Losses from Bleaching (Annual) | $3 Billion (estimated) |
Total Estimated Cost | $20 Billion | Total Estimated Benefit (10 years) | $35 Billion (estimated) |
Outcome Summary
The idea of a calcium sun shield for the Great Barrier Reef is audacious, yet potentially groundbreaking. While the challenges are significant – from design and deployment to environmental impact and cost – the potential rewards are equally immense. Saving this irreplaceable ecosystem requires bold, innovative thinking. The success of this project hinges on a collaborative effort involving scientists, engineers, policymakers, and the global community. The future of the Great Barrier Reef may depend on our willingness to explore and implement such ambitious solutions.